- Title: The International Deep Planet Survey I. The Frequency of Wide-orbit Massive Planets Around A Stars
- Authors: A. Vigan, J. Patience, C. Marois, M. Bonavita, R. J. De Rosa, B. Macintosh, I. Song, R. Doyon, B. Zuckerman, D. Lafrenière, T. Barman
- First author’s institution: School of Physics, University of Exeter
The Big Picture
We’ve written many astrobites about the frequency of planets around Sun-like stars, but what about planets around more massive stars? How common are planets around massive stars? This paper attempts to answer that question by examining the population of planets detected by direct imaging surveys and accounting for the detection sensitivity of the surveys. The authors are launching a broader survey designed to measure the planet occurrence rate across the main sequence from low-mass M dwarfs to massive B dwarfs, but they decided to start with the A stars.
Why Massive Stars?
One of the challenges of directly observing exoplanets is that planets are many orders of magnitude fainter than stars. To put things in perspective, astronomers often liken the task of imaging light from a planet to trying to take a picture of a firefly next to a searchlight. One way to reduce the contrast between the star and planet is to look for planets around less luminous stars, which is why many surveys have attempted to image planets around Sun-like and low-mass stars.
Another strategy is to try to image planets at wide separations from their host stars. The discoveries of the four planets orbiting the A star HR 8799 at projected separations of 68, 38, 24, and 14.5 AU as well as the discoveries of Beta Pictoris b and Fomalhaut b (but see these astrobites) have hinted that gas giants might be common at wide separations from massive stars, so it seems reasonable to design direct imaging surveys to search for planets in distant orbits around A stars.
A second reason to target A stars instead of Sun-like stars is that planets are born bright. A young planet is still cooling off from formation, so the contrast ratio between a young planet and its host star is lower than the contrast ratio between an older, cooler planet and the same star. Since A stars have much shorter lifetimes than Sun-like stars, targeting A stars increases the odds that any planets orbiting the star will be young and bright enough to be detected.
How did they search for planets?
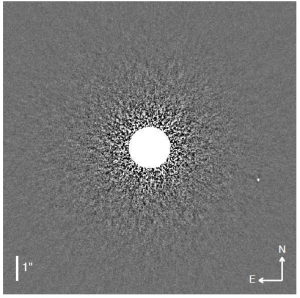
A final image of target star HIP 10670. The point source to the right is a background star. Figure 3 from Vigan et al. 2012.
The authors acquired high-resolution adaptive optics images of 42 young (8-400 Myr), nearby (19-84 pc) A and F stars using two 8 meter telescopes: the Very Large Telescope in Chile and Gemini North in Hawaii. Each of the telescopes is equipped with a special instrument that is optimized for detecting faint planets around bright stars and the authors used a specialized observing strategy known as Angular Differential Imaging (ADI) to spot faint signals from planets that might otherwise be dismissed as scattered light from the star. (See this astrobite for more information about ADI and high-contrast imaging.)
After reducing their data, the authors produce a final image for each star showing the saturated central star and any newly discovered companions. One example is shown at right for the target HIP 10670. Alas, the point source on the right side of the image is a background star and not a planet. The star is 14.6 magnitudes fainter than the primary star, so the authors would have been able to detect planets 14.6 magnitudes fainter (and possibly even fainter) than the target star.
What did they find?
The authors didn’t detect any new planets, but they found three new M star companions to the stars in their survey. They also set upper limits on the masses of planets that could be orbiting their target stars. The authors determined these limits by artificially injecting planets into their unreduced data and seeing how much of the fake planet’s flux was recovered by their data analysis pipeline. They then produced plots of the minimum planet mass that could have been detected as a function of separation from the host star. Since all of their stars have known distances, they can then convert the angular separation in arcseconds to a physical distance in AU (1 AU = the distance from the Earth to the Sun). They use models of young planets to determine the expected luminosities of gas giants at various ages.
In order to convert their individual detection limits for each star into a statement about the occurrence rate of giant planets around A stars, the authors averaged the detection limit of the targets to produce an overall detection limit map. The final detection map is shown in the figure below. The left panel displays a contour map of the probability that a planet would have been detected as a function of planet mass and semimajor axis. The known planets orbiting HR 8799 and Beta Pictoris are overplotted in red and orange. As you can tell from the plot, the survey is most sensitive to planets at separations of 50-400 AU and masses above 10 Jupiter masses. The right panel is another view of the same information. That plot displays the mean probability of detection as a function of semimajor axis for a range of planet masses. The increase in the detection probability as a function of both planet mass and semimajor axis is rather dramatic.
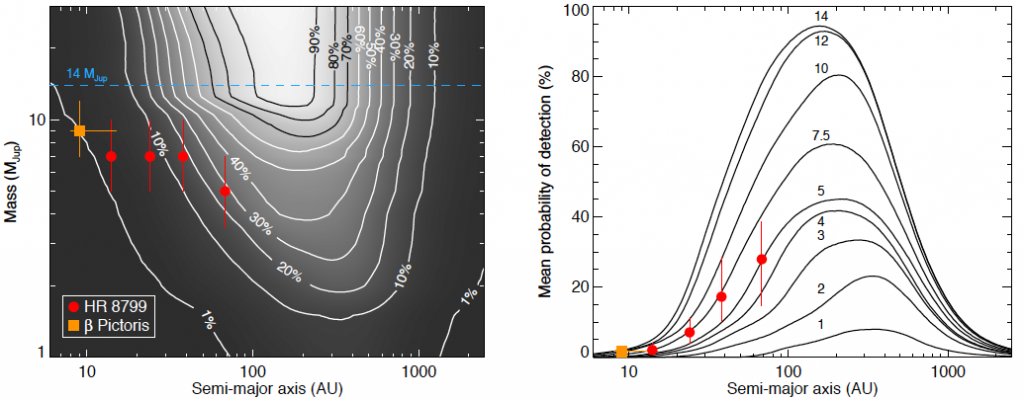
Final detection probability plots for the full sample of stars. Left: Contour map of detection probability as a function of planet mass and semimajor axis. Right: Detection probability versus semimajor axis for different planet masses. Figure 7 from Vigan et al. 2012.
So how common are massive planets around A stars?
Putting everything together, the authors derive an occurrence rate of 8.7% for planets with masses between 3-14 Jupiter masses and separations of 5-320 AU. They also compute the occurrence rate of brown dwarfs (objects with masses of 14-75 Jupiter masses) and discover that 2.8% of A stars host a brown dwarf at a separation of 5-320 AU. Although many of these planets are too faint to be detected by current direct imaging surveys, the authors point out that future surveys using next generation instruments like the Gemini Planet Imager will be able to probe this intriguing population of gas giants.